Openings: Two Theory/Computation Postdoctoral Researchers in Self-Limited Assembly (SLA), UMass Amherst and Brandeis University(1) Computational Soft Matter (Computational modeling of SLA pathways)(2) Theoretical Soft Matter (Theoretical mechanisms and theory-directed design of SLA)
We seek two postdocs to join a multidisciplinary effort connected to the NSF funded Brandeis Bioinspired Soft Materials research center (Ben Rogers, Seth Fraden, Mike Hagan, and Greg Grason), with a primary goal to elucidate the mechanisms of their assembly into self-limiting architectures using theory, computer simulation and experimental study of geometrically programmed “building blocks”. For additional information on the scientific scope of the “Self-Limiting Assemblies” (SLA) project, see below.
We are specifically seeking two postdoctoral positions in theory and computation, one at UMass Amherst hosted in the Grason group, and one at Brandeis University in the Hagan group. Each position will work closely with both Grason and Hagan, as well as experimental groups (Rogers and Fraden), to develop and test controlled SLA systems.
1) Computational modeling of SLA pathways. Location: Brandeis University Qualifications: Experience in computational physics, including molecular dynamics, Monte Carlo, enhanced sampling, data-driven approaches, and/or implementation of large-scale parallelized/GPU-enabled simulations. Tasks: Simulate assembly of finite-sized architectures using molecular dynamics and Monte Carlo approaches. Primary supervisor: Mike Hagan (Brandeis).
2) Theoretical mechanisms and theory-directed design of SLA. Location: UMass Amherst Qualifications: Experience in soft-matter theory, discrete geometry, and computational physics. Applicants are sought with interests in fields such as soft matter, thermodynamics, and materials science. Tasks: Develop geometrical principles to design new SLA ‘’building blocks ». Develop theoretical models of emergent behavior of geometrically frustrated assembly. Primary supervisor: Greg Grason (UMass: Amherst).Both positions offer ample opportunities for your professional development including participating in exciting cutting-edge science, gaining mentoring experience, and initiating your own research directions. Women and minority candidates are encouraged to apply. UMass Amherst and Brandeis University are Affirmative Action/Equal Opportunity employers.
Start Date: As soon as September 2020.
Submit applications to both grason@mail.pse.umass.edu and hagan@brandeis.edu specify which position(s) you are applying for. Please include a CV, a list of references, and a brief description of your previous research.
MRSEC collaboration on “Self Limiting Assemblies”:
The SLA collaborative team (Rogers, Fraden, Hagan and Fraden) will develop a versatile class of DNA-origami building blocks to elucidate the fundamental physical principles for engineering components that self-assemble into large, but finite-size, superstructures. The self-assembly of size-controlled architectures is prevalent in living systems. The adaptive functions of biological materials, including viral shells, cytoskeletal filaments, and photonic nanostructures of bird feathers arise from the regulated finite size of self-assembled architectures. In contrast, most inorganic materials form unlimited structures like crystals. In this project, we will advance two complementary paradigms for bottom-up assembly of size-controlled architectures. One uses curvature programmed building blocks that assemble into self-closing structures (e.g. tubules and capsules). The other advances a new paradigm of geometrically-frustrated assembly, in which ill-fitting blocks accumulate stresses that grow with assembly and limit their thermodynamic size.
This collaborative research addresses many fundamental questions in self-assembly: How do shapes, interactions, and flexibilities of building blocks control the assembly size? How can self-limiting assembly be adapted to distinct morphologies, like ropes, fibers, sheets or shells? Are there fundamental or practical limits to the sizes of controllably assembled structures? By answering these questions and more, we aim to develop engineering principles to create size-controlled architectures with high yield, with the structural control currently only possible via “top down” fabrication techniques (e.g. 3D printing and lithographic fabrication).
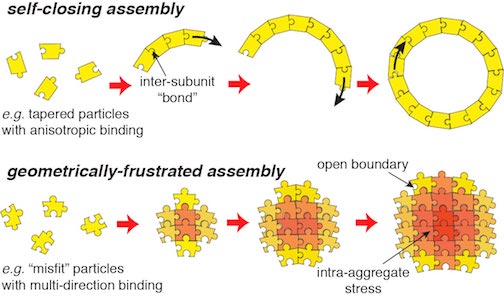